Marvellous Medical Materials: Stainless Steel - Part One
08 November 2017
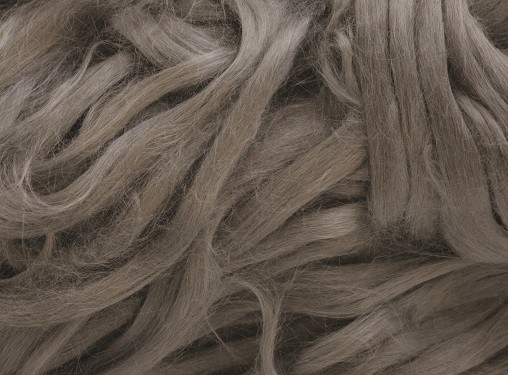
I began writing this blog post having just got back from the International Agatha Christie Festival where I spent the weekend talking about murderous materials with fellow Poirot fans, so the dynamic relationship between poisonous and medicinal materials was very much at the forefront of my mind.
In particular, I’d been thinking about stainless steel and its relationship to health: in terms of the occupational risks of producing and working with it as well as its many beneficial medical uses. In the first part of this three part blog post, I explore the gradual historical domination of stainless steel in surgical tools and medical implants.
Stainless steel is so-called because of its corrosion-resistance, a property largely afforded by the addition of chromium (Cr) to the alloy. This chromium content reacts with oxygen to form a very stable chromium oxide bond at the surface, which means it does not rust. Nickel (Ni) is also well-known for being a key ‘stainless metal’, and plays a crucial role in the production of austenitic stainless steels where it gives these non-magnetic grades their strength, ductility and toughness as well as making them more inert inside the body (Street and Alexander 1994; Kirkup 1993).
As a result of these properties, stainless steel has become a stalwart of the modern hospital. Various grades of this material are used in a wide range of medical applications, from the mundane to the specialized, for everything from hospital beds and handrails to hip replacements, prosthetics and sterilisable surgical instruments. This material domination is relatively new though: a much wider palette of materials used to be common in hospitals.
Early 19th century stethoscopes, for example, like the one pictured below, were often made from the same finely grained and lightweight woods used for wind instruments (e.g. boxwood and fir). And before the introduction of thermal sterilization (between 1885 and 1910), when surgery was conducted without asepsis (or anaesthetic!) (Fitzharris 2017), surgical instruments were commonly made using ivory, tortoiseshell, wood, copper, bronze, iron, silver and gold (Gugliemino et al. 2016).
Thermal sterilisation revolutionised the surgeon’s armoury: it proved destructive to the organic materials on instruments (e.g. bacteria, blood) but also to the wood, ivory and tortoiseshell used to make these tools (Kirkup 1993). All-metal instruments became the norm, and because thermal sterilisation accelerated steel corrosion, these were further protected by plating them with nickel and chrome. However, these plated steels still had the tendency to peel, flake and rust underneath: a problem eventually eliminated by the introduction of stainless steel in the early 20th century. Between 1913 and 1925 stainless steel gradually superceded most other materials in surgical instruments.
Having discussed the surgical advances and new hygienic environments that stainless steels made possible, the next part of this blog post turns to the negative effects of steel on the human body. Nickel and chromium, the constituent ingredients of stainless steel, are both known carcinogens, and nickel also has the dubious honour of having been voted ‘Allergen of the Year’ in 2008 by the American Contact Dermatitis Association. So why do people use stainless steels for medical purposes if their component parts have this potential to cause adverse health effects?
Well, one of the central principles of toxicology is that the effects of a material on the human body depend on the form of the material, the volumes we are exposed to, and the context in which we encounter them. The risk from nickel, for example, largely comes from encountering it in its raw form: once alloyed with steel it presents little risk to the consumer encountering it in everyday use.
The health effects of chromium also depend on the form in which we encounter them: whilst the high levels of toxicity and carcinogenic properties of hexavalent chromium (Cr+6) have been known about since the late 1800’s (Antonini 2003), metallic chromium (Cr) and trivalent chromium (Cr+3) (the same substance in different oxidation states) are both of a low order of toxicity and are found in trace amounts in food and water. The chromium that most patients and consumers will encounter in finished stainless steel in surgical tools and the hospital environment is not hazardous.
In its finished form, stainless steels is relatively inert, strong, corrosion-resistant and durable, so it is understandable that it has become one of the materials of choice for use inside the body. Before the advent of stainless steel, a wide variety of metals like gold, brass, aluminium, platinum and nickel-plated steels were used for dentistry, prostheses and to fix fractures, but most of these were later found to be unstable with a tendency to corrode or leach into the body (Agrawal 1998). Materials implanted in the body are in continuous or intermittent contact with bodily fluids, so have to be corrosion-resistant, biocompatible, nontoxic and non-carcinogenic, and in the case of a joint prosthesis for example, have the physical and mechanical properties to withstand heavy loads and strains. These stringent requirements eliminate all but a few engineering materials. For this reason, from the 19th century onwards corrosion-resistant metals like stainless steel and titanium came to displace all other metals for in vivo applications, and stainless steel is still one of the most frequently used materials for fracture fixation.
However stainless steel is not suitable for all biomedical applications. This is because the health effects of a biomaterial are not just about the interaction between one material and the human body: interactions between different materials or components also have to be considered. Stainless steel was used in a variety of early metal-on-metal hip implant designs between the 1930s and 1950s (Reynolds & Tansey 2007), but it is now known that the abrasive action of the two parts of the steel hip joint rubbing together resulted in the release of toxic metal ions into the body. Equally, the metal-on-plastic (stainless steel and PTFE) implants developed in the 1960s released harmful metal and plastic wear debris into the body. The long-term physiological effects are still not entirely understood, but the metal debris in particular includes nanoparticles of chromium (Cr), cobalt (Co), nickel (Ni) and iron (Fe). These particles accumulate locally causing allergic reactions that can lead to infections, the failure of the implant and tumours (Schaffer et al. 1999; Keegan et al. 2008). Because these metal particles are so small they are thought to be more toxic and to travel around the body interfering with the a variety of wider biological functions including the vascular system, immune system, reproductive function and DNA repair. This is not a new problem, and stainless steel is not the only problematic material: there have since been concerns about the body’s reaction to a variety of structural biomaterials, including cobalt-chromium alloys, titanium and polyethylene (PTFE). The debate about the best material for these kinds of implants still continues.
Stainless steel’s harmful effects can also be seen in its industrial production, where it is by no means a docile material. As well being subject to all the usual risks of hot and noisy metal work, stainless steels welders are at a higher than normal risk of respiratory illnesses like occupational asthma, bronchitis, metal fume fever and lung cancers (Simonato et al. 1991, Hansen et al. 1996, Antonini 2003). Vaporized chromium, nickel, manganese, zinc oxide, iron and silica present in the welding fumes are thought to be the cause of these particular occupational health hazards. Hexavalent chrome also manifests itself during the hot work of production.
Even a very brief survey of the history of pharmaceuticals highlights the fact the same substance can be considered both a poison and a medicine. Radon, for example, can be both a toxin, as a major cause of lung cancer, and a medicine, as a gas used therapeutically for its analgesic and anti-inflammatory effect (Erickson 2007). The materials that we consider to be medicinal or harmful also vary cross-culturally and historically. Arsenic, for example, one of Agatha Christie’s poisons of choice, was used medicinally by early Chinese healers as a bacteriocide (Hulse 2004), by the Greeks for hair removal and the treatment of ulcers, and by the Victorians as a cure-all ‘therapeutic mule’ (Bentley & Chasteen 2002) used to treat everything from malarial fever, skin disease, neuralgia and even finding its way into everyday hand soap.
The same is true of materials like stainless steel and silicone rubber: their health and societal impacts are not just the result of a fixed set of physical properties, but depend on how and where we use those materials, and in what volumes. Substances that are known to be hazardous in some forms, contexts and volumes can be useful and protective in others. Although it has been banned for use in most applications by the European Chemicals Agency as a substance of very high concern (SVHC), hexavalent chrome is still authorised for use by the aerospace and automotive industries. Because of the unparalleled hardness, shininess and corrosion resistance it gives alloys, it is still used in the as a primer for parts whose failure could have a catastrophic impact on the safety of the car or plane passenger (Wilkes 2014). This known carcinogen can have an indirect and positive impact on human wellbeing.
In thinking through the ways in which stainless steel influences human health and wellbeing this blog post demonstrates that the same material can be both poison and panacea. The health of a material is not just a question of its physical properties and effects on the human body; it is also related to the material’s applications and societal benefits. This post also shows that the perceived health of a material is dynamic, and is as much a question of politics and ethics as it is one of toxicology and materials science: depending as it does on culturally- and historically-specific understandings of toxicology and levels of acceptable risk.
References
Agrawal, C. M. (1998). Reconstructing the human body using biomaterials. Journal of the Minerals, Metals and Materials Society, 50(1), 31-35.
Antonini, J. M. (2003). Health effects of welding. Critical reviews in toxicology, 33(1), 61-103.
Bentley, R., & Chasteen, T. G. (2002). Arsenic curiosa and humanity. The Chemical Educator, 7(2), 51-60
Erickson, B. E. (2007). Toxin or medicine? Explanatory models of radon in Montana health mines. Medical anthropology quarterly, 21(1), 1-21.
Fitzharris, L. (2017). The Butchering Art: Joseph Lister's Quest to Transform the Grisly World of Victorian Medicine. Scientific American/Farrar, Straus and Giroux.
Gugliemino, B., Lake Thomas, Z., Curtis, B., Baranow, S. and Lotzof, K. 2016. Robert Liston’s Surgical Instruments. UCL Collection Curatorship Project. Available at: https://www.academia.edu/30768024/Robert_Listons_Surgical_Instruments_UCL_Scientific_and_Engineering_Collections_Research
Hansen, K. S., Lauritsen, J. M., and Skytthe, A. (1996). Cancer incidence among mild steel and stainless steel welders and other metal workers. Am. J. Ind. Med. 30:373–382.
Haudrechy, P., Mantout, B., Frappaz, A., Rousseau, D., Chabeau, G., Faure, M., & Claudy, A. (1997). Nickel release from stainless steels. Contact Dermatitis, 37(3), 113-117.
Hulse, J. H. (2004). Biotechnologies: past history, present state and future prospects. Trends in food science & technology, 15(1), 3-18.
Kanerva, L., Sipiläinen‐Malm, T., Estlander, T., Zitting, A., Jolanki, R., & Tarvainen, K. (1994). Nickel release from metals, and a case of allergic contact dermatitis from stainless steel. Contact Dermatitis, 31(5), 299-303.
Keegan, G. M., Learmonth, I. D., & Case, C. (2008). A systematic comparison of the actual, potential, and theoretical health effects of cobalt and chromium exposures from industry and surgical implants. Critical reviews in toxicology, 38(8), 645-674.
Kirkup, J. (1993). From flint to stainless steel: observations on surgical instrument composition. Annals of the Royal College of Surgeons of England, 75(5), 365.
Reynolds, L. A., & Tansey, E. M. (2007). Early development of total hip replacement. Wellcome Trust Centre for the History of Medicine at UCL.
Schaffer, A. W., Schaffer, A., Pilger, A., Engelhardt, C., Zweymueller, K., & Ruediger, H. W. (1999). Increased blood cobalt and chromium after total hip replacement. Journal of Toxicology: Clinical Toxicology, 37(7), 839-844.
Simonato, L., Fletcher, A. C., Andersen, A., Anderson, K., Becker, N., Chang-Claude, J., ... & Hansen, K. S. (1991). A historical prospective study of European stainless steel, mild steel, and shipyard welders. Occupational and Environmental Medicine, 48(3), 145-154.
Street, A., & Alexander, W. (1994). Metals in the Service of Man. Penguin Books.
Wilkes, S. (2014). In Search of Sustainable Materials: Negotiating Materiality and Morality in the UK Materials Industry. PhD Thesis, University College London.